“Bio-structural” Erosion Control: Incorporating Vegetation In Engineering Designs To Protect Puget Sound Shorelines
Presented February 13, 2001 at Puget Sound Research 2001, a conference relating to the Puget Sound/Georgia Basin Ecosystem in Bellevue, WA. Sponsored by the Puget Sound Water Quality Action Team.
Abstract
The conventional engineering approach to slope stabilization and erosion control usually relies solely on structural components. Vegetation is rarely included in engineering designs, though occasionally it is treated as incidental landscaping. Though the benefits of vegetation's role in erosion control are poorly understood within the engineering community; the value of vegetation in controlling erosion and reducing shallow mass wasting is well documented.
While engineered structures provide immediate stabilization and erosion abatement, they become progressively weaker over time and do not adapt to changing site conditions. Vegetation, though ineffective when first established, becomes progressively more effective, adaptable, and self-perpetuating over time. Vegetation also improves water quality, reduces storm water run-off, enhances wildlife and fisheries habitat, improves aesthetics, and reduces noxious weed establishment.
A “Bio-Structural” approach to erosion and slope stability problems; i.e., incorporating planned vegetational elements in engineering designs, can be less expensive, more effective, and more adaptable than purely structural solutions. Vegetation should be used in conjunction with geo-textiles and engineered structures whenever appropriate and practical.
Vegetation selected for “Bio-structural” design elements should be native whenever possible. Plants chosen should also be appropriate to the site, have wide adaptability, favorable spread and reproductive capability, superior control value, roots of high tensile strength, and be available commercially.
Author Biography
Elliott Menashe, of Greenbelt Consulting on Whidbey Island, has been a natural resource management consultant since 1987. He received a degree in Forest Management from the University of California at Berkeley in 1975. His firm specializes in marine shoreline, forest, and riparian management, as well as low-impact rural development practices, and restoration of degraded sites. He can be reached at (360) 341-3433. Email: elliott@greenbeltconsulting.com
I. Introduction
Surface erosion and mass soil losses from landslides are of great concern to land managers. Accelerated erosion and slope instability can be caused or exacerbated by human activities. Increased erosion can cause adverse cumulative watershed problems by increasing sedimentation, degrading water supplies, reducing forest productivity, destroying anadromous fish habitat, and degrading other critical environmental functions. Mature structurally and floristically complex plant communities significantly reduce surface erosion and contribute greatly to maintaining slope stability. Management of forested, coastal, urban, agricultural, and riparian areas should conserve plant cover. The relative effectiveness of vegetation in any specific locale will be a function of site-specific conditions.
The conventional engineering approach to slope stabilization and erosion control usually relies solely on structural components. Vegetation is rarely included in engineering designs, though occasionally it is treated as incidental landscaping. Though the benefits of vegetation’s role in erosion control are poorly understood or appreciated within the engineering community, the value of vegetation in controlling erosion and reducing shallow mass wasting is well documented. The use of vegetation and biotechnical measures should be incorporated into engineering designs early in the planning and design phases of a project.
II. Role of Vegetation
“Vegetation affects both the surficial and mass stability of slopes in significant and important ways.” “The stabilizing or protective benefits of vegetation depend both on the type of vegetation and type of slope degradation process. In the case of mass stability, the protective benefits of woody vegetation range from mechanical reinforcement and restraint by the roots and stems to modification of slope hydrology as a result of soil moisture extraction via evapotranspiration.” (Gray and Sotir, 1996).
“The loss or removal of slope vegetation can result in either increased rates of erosion or higher frequencies of slope failure. This cause-and-effect relationship can be demonstrated convincingly as a result of many field and laboratory studies reported in the technical literature.” (Gray and Sotir, 1996). Vegetation also improves water quality, reduces storm water run-off, enhances wildlife and fisheries habitat, improves aesthetics, and reduces noxious weed establishment.
1. Benefits of Vegetation in Preventing Surficial Erosion
Protocols have been developed to describe the factors that are instrumental in vegetation’s effectiveness in limiting surface erosion. Wischmeier (1975) identified three major sub-factors: (I) canopy, (II) surface cover, and (III) below surface effects. Dissmeyer and Foster (1984) modified and made additions to the earlier work to adapt it to forest conditions. The basic forest sub-factors useful in applying the modified universal soil loss equation (USLE) include ground cover, canopy, soil reconsolidation, organic content, fine roots, residual binding effect, and on-site storage of water.
Gray and Leiser (1982) provide a summary of the major effects of herbaceous and woody vegetation in minimizing erosion of surficial soils. They include:
- Interception – foliage and plant residues absorb rainfall energy and prevent soil compaction.
- Restraint – root systems physically bind or restrain soil particles while above-ground residues filter sediment out of run-off.
- Retardation – above-ground residues increase surface roughness and slow run-off velocity.
- Infiltration – roots and plant residues help maintain soil porosity and permeability.
- Transpiration – depletion of soil moisture by plants delays onset of saturation and run-off.
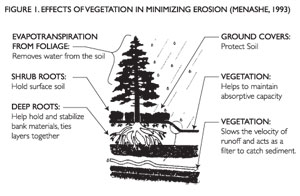
Greenway (1987) notes that “roots reinforce the soil, increasing soil shear strength”, “roots bind soil particles at the ground surface, reducing their susceptibility to erosion,” and “roots extract moisture from the soil…, leading to lower pore-water pressures.” Several layers of vegetation cover, including herbaceous growth, shrubs, and trees, multiply the benefits discussed above. (Figure 1).
2. Benefits of Vegetation in Slope Stabilization
A substantial body of credible research concerned with vegetation and slope stability exists. Most of the literature supports the contention that, in the vast majority of cases, vegetation helps to stabilize a slope (Macdonald and Witek, 1994). As Gray and Leiser (1982) remark, “The neglect of the role of woody vegetation (and in some instances its outright dismissal) in stabilizing slopes and reinforcing soils is surprising.” Their summary of beneficial influences of woody vegetation follows:
- Root Reinforcement – roots mechanically reinforce a soil by transfer of shear stresses in the soil to tensile resistance in the roots.
- Soil moisture modifications – evapotranspiration and interception in the foliage limit buildup of soil moisture stress. Vegetation also affects the rate of snowmelt, which in turn affects soil moisture regime.
- Buttressing and arching – anchored and embedded stems can act as buttress piles or arch abutments in a slope, counteracting shear stresses.
Gray and Sotir (1996) added a fourth beneficial effect. (The earlier work listed it as potentially negative).
- Surcharge – weight of vegetation can, in certain instances, increase stability via increased confining (normal) stress on the failure surface.
The degree to which vegetation stabilizes slopes and reinforces soils in any specific locale is enormously complex. Several analytic models that attempt to quantify controlling factors have been developed. (Sidle, et. al., 1985).
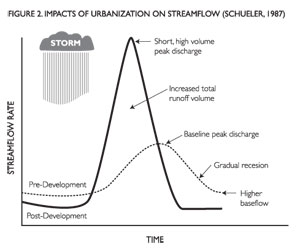
Greenway (1987) notes “that as vegetation is removed from a watershed, water yield increases and water table levels rise”. Permanent loss of vegetation cover, or replacement by ineffective vegetation, increases soil saturation and surface water run-off. Vegetated watersheds exhibit lower peak flows, lower total discharge volumes, and increased lag-time between rainfall and run-off than do watersheds where effective vegetation has been removed. (Figure 2).
3. Limitations of Vegetation
While undisturbed mature native vegetation on slopes provides erosion control and slope stabilization benefits, disturbed or degraded sites undergo continual erosion, and may not establish an effective cover. Vegetation alone may be relatively ineffective where hydrologic influences, fluvial processes, or wave attack repeatedly interrupts natural plant succession and favors less effective species. Competition by invasive, exotic plants such as Himalayan blackberry can also retard or preclude natural establishment of effective vegetation. Hydro-seeded grasses are often ineffective in minimizing surface erosion subsequent to construction and additional expenditures are necessary to repair slopes damaged by rills and gullies. Grass provides virtually no slope stabilization benefits. Grassed slopes provide negligible storm water filtration benefits compared to native ground covers. Grasses are ineffective in discouraging the establishment of undesirable invasive plants.
Vegetation alone is ineffective in the presence of deep-seated instability and active mass wasting. A disturbed or modified site must be stable enough to allow establishment and development of an effective plant community, often for as long as 10 years.
III. Engineered Measures Provide Stabilization, But At A Cost
Where accelerated erosion, slope destabilization, and landslides have occurred, engineered measures suited to the geomorphologic conditions are often necessary to stabilize the site. Engineering solutions aim to both reduce the influences of destabilizing forces and physically arrest slope failure and surface erosion. There are four basic methods used to improve slope stability:
- Unloading the head of the slope
- Ground and surface water regime modification
- Buttressing the toe of the slope
- Shifting the position of the potential failure surface
The specific measure or combination of measures employed is dependent upon a wide variety of complex factors, including geomorphology, hydrology, slope, climate, failure type, and topography. Macdonald (1994) provides an excellent written and photographic description of commonly employed conventional structures and hydrologic control measures in the Puget Sound region. Most engineered solutions result in significant incidental slope modification and environmental impacts. Toe stabilization on marine and riparian shorelines, such as riprap, are disruptive to nearshore habitat and affect coastal processes. Slope stabilizing measures, such as stepped crib walls, change slope geometry. Drainage measures, such as horizontal drain piping, alter both slope and down-gradient hydrology.
While engineered solutions effectively provide immediate stabilization and erosion abatement, they also cause environmental impacts to public resources. Removal of vegetation is common during construction of structures. Loss of vegetative cover often initiates soil degradation causing the site to become less productive. Conventional erosion control and revegetation efforts subsequent to construction are often ineffective and fail to adequately protect bare soil from incidental surface erosion and adjacent slope impacts. Products such as “jute” mats are ineffective in reducing surface erosion or encouraging the establishment of effective vegetation.
Engineering measures deteriorate over time, becoming progressively less effective or failing entirely. Adjacent slope movement can involve structures and impair their effectiveness. Where revegetation efforts consist merely of hydro-seeding or sod, ineffective vegetation is likely to become established, providing few of the benefits discussed above. If desirable effective vegetation is not deliberately incorporated into engineered measures, slope problems may become recurrent over the long term.
IV. Bio-Structural Approach
A Bio-Structural approach to erosion and slope stability problems (i.e., incorporating planned woody vegetational elements in engineering designs) can be less expensive, more effective, and more adaptable over the long term than purely structural solutions. Revegetation and biotechnical measures should be used in conjunction with geotextiles and engineered structures whenever appropriate.
Bio-structural erosion control and slope stabilization includes the measures known as soil bioengineering and biotechnical slope protection. As Gray and Leiser (1982) state, “both biological and mechanical elements must function together in an integrated and complementary manner”. Gray and Sotir (1996), refer to soil bioengineering “as a specialized area or subset of biotechnical stabilization”. The bio-structural approach is not new. McCullah (1996) quotes an anonymous wiseman from the California Division of Highways (1950), “The most successful erosion control methods have proved to be those which reproduce most closely conditions which are found on natural slopes. Mechanical or unnatural methods of control, while sometimes immediately effective, deteriorate with time and show up poorly in the long run as compared with methods that follow natural vegetative processes. If we work with nature, erosion control problems are simplified and the probability of success becomes more certain than if we disregard the examples of successful natural stabilization to be found on every hillside…”.
Several excellent manuals relating to theory and general practical applications of biotechnical measures are available. Gray and Leiser (1982) include an annotated bibliography. Schiechtl (1980) details bioengineering research and practice in Europe. Gray and Sotir (1996) include descriptions of recent advances in geotextiles and mechanical measures. The following is a very brief summary of important factors to consider when incorporating planting and biotechnical measures in engineering designs.
1. Define Objectives
What do you hope to achieve by incorporating vegetation in an engineering design? Some common objectives and goals include the following:
- Erosion control (rilling and gullying)
- Slope stabilization (marine, riparian, terrestrial)
- Restoration of pre-project vegetative cover
- Creation of wildlife and fisheries habitat (cover, food, and shade)
- Stormwater management (reduction of run-off and sedimentation)
- Aesthetic enhancement (landscape restoration)
- Regulatory mitigation (buffer enhancement)
- Reducing invasive plant establishment
2. Suitability of the Site
What are the physical environmental, and social characteristics of the site? Is revegetation possible and desirable? Each site is different and unique. Failure to consider pertinent factors often results in failure of biotechnical and planting efforts.
General Physical Characteristics:
- Topography
- Soils
- Slope
- Hydrology
- Aspect
- Geomorphology
- Climate
General Environmental Characteristics:
- Wind
- Salt (spray, tidal)
- Soil moisture and productivity
- Sun/shade conditions
- Precipitation (rain, snow, fog)
- Presence of invasive exotic plants
- Flooding and/or inundation
- Potential animal impacts
Social Considerations:
- Offsite influences (drainage, invasive plants)
- Land use regulations
- View constraints
- Conflicting objectives (view vs. erosion control)
3. Project Design
It is imperative that planting and biotechnical measures be incorporated into the design from the project’s inception. Vegetation should be considered integral to design rather than incidental. A team approach from first reconnaissance and feasibility through final construction will assure a successful project. Vegetational and engineering measures need to be coordinated to be effective. Common components of such projects may include structural, geotextile, biotechnical, and planting measures. Communication between project team members will minimize disruption to construction schedules and prevent other potential problems. Installation of vegetational measures often needs to be coordinated with mechanical structures and groundwork efforts. This is especially important where riprap or other slope-face stabilization measures are planned.
4. Vegetation Component of Design
Every effort should be made to understand the specific constraints and opportunities of the site and project. Reference sites adjacent to the project should be surveyed to identify desirable species and plant communities for erosion control, slope stabilization, and wildlife and fisheries habitat value. If bioengineering measures are to be used; survey local areas for suitable plant materials for cuttings. Note any significant disease or insect problems. Determine if undesirable plant seeds will be a problem if existing project topsoil is to be used. Mulch or geotextile may be needed to reduce plant competition with new plantings. There are no “cookbook” plant lists or generic solutions. An inappropriate plant or biotechnical measure in the wrong place will compromise the project’s effectiveness and waste money. Micro-site factors may need to be considered on project sites with varying slope, aspect, hydrology, and soils. All the factors listed previously regarding physical, environmental, and social characteristics should be specifically considered in plant and biotechnical measure selection.
Species selected should have the following attributes:
- Native to the area
- Appropriate to the site (e.g. salt tolerant, drought hardy)
- Have a wide biologic amplitude of adaptability
- Favorable spread and reproductive capability
- Superior erosion control value
- Excellent root spread and strength
- Be commercially available in adequate numbers or able to be contract-grown (1-2 year lead time).
Plant materials are available in a variety of stock types. Use of cuttings, bare-root stock, planting tubes, containers, or other types are all common. The type of plant stock selected will be dependent on various project-specific factors. These include planting season, site characteristics, plant availability, and soil type. Seeding of native woody vegetation is seldom practical or effective.
5. Additional Planning Issues
Site preparation is a crucial element in any planting or biotechnical project. Eradication of undesirable species from the planting site and topsoil seed bank is critical. On sites with harsh exposures or droughty sites, irrigation may be required. The use of geotextile fabric may provide multiple benefits, including immediate erosion control, control of competing vegetation, and conservation of soil moisture. Animal damage protection for new plantings is often necessary to reduce losses.
6. Monitoring, Maintenance, and Replacement
Many planting and biotechnical projects fail from neglect. Vegetative measures require care during the establishment period, from one to three years after installation. Contingency plans, and funds to implement them, should be part of project specifications. Vegetation measures are weak, ineffective, and vulnerable when first installed, but become progressively stronger, more effective, more adaptable, and self-perpetuating over time. If proper establishment, monitoring, and maintenance measures are undertaken subsequent to installation, the site should be self-sufficient after the third year.
Some Monitoring Elements to Assess Include:
- Mortality (replace dead plants)
- Damage (animal, insects, disease, vandalism)
- Wilting (check soil moisture regime)
- Trampling (human, animal)
- Adequate growth (to achieve coverage and effectiveness)
- Competing vegetation (control or eradication indicated)
- Erosion or hydrologic damage
Important Maintenance Efforts Include:
- Replant as necessary to maintain stocking
- Irrigate as necessary
- Remove undesirable competing vegetation
- Protect plants from animal damage (browsing, trampling, etc.)
V. Conceptual Description of a Soft-Shore Alternative To Marine Bulkheads and Revetments: The “Root Wall”
The root wall concept is proposed to provide a bio-structural alternative to conventional marine bulkheading. The root wall represents an innovative, environmentally acceptable form of shore protection that minimizes adverse impacts common to conventional shore armoring measures. It will also significantly improve nearshore habitat features and provide for complex shoreform creation. A root wall will mimic naturally occurring accumulations of marine driftwood, which protect shorelines and prograde beaches.
The root wall concept has been developed by Elliott Menashe of Greenbelt Consulting, in collaboration with Jim Johannessen of Coastal Geologic Services. Preliminary feasibility and engineering design work has been provided by Theodore Hammer of Western Geotechnical Consultants. While the use of large woody debris has been a common practice in stream restoration for decades, marine applications have not yet been attempted as described herein.
1. Root Wall Conceptual Description
The root wall employs large tree root masses, trunk and root masses, and other large woody debris (LWD) as primary structural components to provide immediate toe protection and bluff stabilization. LWD to be used as structural components exposed to wave attack would consist of durable tree species resistant to rot and abrasion. Drift logs without attached root masses are of minimal value because they are too mobile and difficult to anchor to be of value.
The planting, establishment, and development of trees and shrubs behind the structure are integral to the root wall system’s design. Incorporating planned vegetation elements in the engineering design provides short-term and long-term erosion control, as well as environmental benefits. Incorporation of vegetation in the design provides long-term structural reinforcement of components through root matrix development. Vegetative components become more effective, adaptable, and self-perpetuating over time, while conventional bulkheads are strongest when built but progressively weaker and more prone to failure over time. Trees planted as project components will be gradually recruited as additional shore protection. Shrub and ground covers will reduce surface erosion and filter sediments. This approach mimics well-documented naturally occurring processes.
The strength of the root wall would be achieved by interlocking component pieces to allow flexibility without compromising structural integrity. Major components would be partially buried in beach substrate and further secured by backfill to resist movement caused by wave action. The composite structure would be anchored into the underlying substrate as necessary.
The root wall concept can be used in conjunction with other soft-shore protection measures, such as beach nourishment. Potential sites for the root wall include low-to-moderate wave energy beaches and areas sporadically subject to wave attack. The root wall approach would be ideal where restoration of marine uplands is a critical objective.
2. Benefits of the Root Wall Over Conventional Hard-Structures:
Physical
The root wall would reduce reflection and refraction of wave energy thereby reducing sediment suspension, “scour”, and transport. The root wall would also reduce “end wall” erosion. Large woody debris would buttress the toe of the slope as well as reduce up-slope sediment runout onto the beach. A more stable angle-of-repose would be achieved. Additional floating LWD would be captured during high-water storm events. The energy of waves would be dissipated and their destructive impacts on the shore would be minimized. Groundwater discharge would be unimpeded and hydrologic connectivity between uplands and beach would be maintained. The root wall would create complex shoreforms and microhabitat features.
Biological
The root wall would provide crucial habitat features as integral structural components. LWD supports a large number of biological functions and linkages. The root wall would encourage rapid naturalization of the backshore and lower bluff. Nearshore habitat features, such as overhanging vegetation and well-shaded foreshore areas, would be encouraged. Fish habitat in the nearshore would also be improved by increased introduction of organic matter into the marine system, and by enhanced refuge from predators, movement corridors, and food sources.
Additional Benefits
The root wall would improve nearshore and distant-view aesthetics. Within ten years, a well-constructed and planted root wall would appear to be a natural feature. Large woody material providing the structural components are virtually free, except for the cost of transportation to the site. Short-term construction impacts would be similar to those of conventional structures. Unlike conventional structures, the mid-and long-term effects of the root wall would be a significant improvement of the shoreline. The root wall and vegetative components would comprise a self-perpetuating system for long-term shore protection and naturalization.
VI. Conclusion
Extensive clearing, grading, and slope modification are concomitant impacts of conventional erosion control and slope stabilization projects. Revegetation measures are often only an incidental component and are inadequate or ineffective, leading to the establishment of undesirable, invasive exotic plants subsequent to construction. Sedimentation of drainage facilities and adverse impacts to water quality, as well as degradation of fish habitat, are often unintended consequences. Existing mechanical best management practices and engineered hydrologic controls can be ineffective in mitigating increased and cumulative storm water impacts in a watershed. Critical area buffers are often inadequate protection for headwater streams and wetlands in the upper reaches of a watershed. Marine shorelands and nearshore habitats are adversely impacted by increased development and the proliferation of bulkheads and other shore protection structures.
The recent listing of several salmonid species under the Endangered Species Act has focused attention on the importance of maintaining effective, native vegetation cover and minimizing impervious surfaces. The extent of hidden environmental, economic, and social costs of urbanization are becoming evident and alarming.
If native, woody vegetation planting and successful establishment becomes a routine objective of engineering plans and projects, then many of the adverse impacts and effects noted above will be significantly reduced.
Potential applications include slope stabilization, road and right-of-way, marine shore protection, and stream projects. Restoring the most valuable and effective plant communities on construction sites would also reduce future maintenance costs, reduce long-term erosion and landslide rates, improve wildlife and fish habitat, improve water quality, and help to maintain the aesthetic features synonymous with our region. While individual projects may have a relatively small benefit, the cumulative beneficial impacts are potentially enormous.
References
- Burroughs, T. and C. Weller, 1996. Linking Land Use and Water Quality: How Development and Stormwater Runoff Affect Our Streams, Rivers, Bays, and Lakes. Prepared for: 1000 Friends of Washington, Seattle, WA.
- California Division of Highways. 1950. Erosion Control On California State Highways. Dept. of Transportation, Sacramento, California.
- Chatwin, S. C., et al. 1991. A Guide to Management of Landslide-Prone Terrain in the Pacific Northwest. Land Management Handbook Number 18. B.C. Ministry of Forests, Victoria, B.C., Canada.
- Connin, S. 1991. Characteristics of Successful Riparian Restoration Projects in the Pacific Northwest. EPA-910/9-91-033. Water Division, Environment Protection Agency, Region 10, Seattle, Washington.
- Daubenmire, R. F. 1947. Plants and Environment: A Textbook of Plant Autecology. Wiley and Sons. New York, New York.
- Dissmeyer, G. E., and G. R. Foster. 1981. Estimating the Cover – Management ( C ) in the Universal Soil Loss Equation for Forest Conditions. Journal of Soil and Water Conservation. 36 (4):235-240.
- Dissmeyer, G. E., and G. R. Foster. 1984. A Guide for Predicting Sheet and Rill Erosion on Forest Land. U.S.D.A. Forest Service, Southern Region, Atlanta, Georgia.
- Franklin, J. F. and C. T. Dyrness. 1973. Natural Vegetation of Oregon and Washington. GTE-PNW-8. PNW Research Station, USDA, Forest Service, Portland, OR.
- Fredricksen, R. L., and R. D. Harr. 1981. Soil, Vegetation and Watershed Management. In Forest Soils of the Douglas Fir Region. P. E. Heilman, H. W. Anderson, D. M. Baumgartner (editors) Washington State University Co-op Extension Service.
- Gray, D. H., and A. T. Leiser. 1982. Biotechnical Slope Protection and Erosion Control. Van Nostrand Reinhold Company. New York.
- Gray, D. H., and R. B. Sotir. 1996. Biotechnical and Soil Bioengineering Slope Stabilization: A Practical Guide for Erosion Control. John Wiley and Sons.
- Greenway, D. R. 1987. Vegetation and Slope Stability. In Slope Stability, edited by M. F. Anderson and K. S. Richards. Wiley and Sons, New York.
- Johannessen, J. 2000. Alternatives to Bulkheads in the Puget Sound Region: What is Soft Shore Protection & What is Not? In: Proceedings of Coasts at the Millenium, Coastal Society’s 17th International Conference. July 2000. In Press.
- Juelson, T. C. 1980. Suggestions for Stream Bank Revegetation in Western Washington. Habitat Management Division, Washington Department of Game, Olympia, WA.
- Kittredge, J. 1948/1973. Forest Influences: The Effects of Woody Vegetation on Climate, Water, and Soil, With Applications to the Conservation of Water and the Control of Floods and Erosion. (1973) Dover Publications, New York.
- Macdonald, K. B., and B. Witek. 1994. Management Options for Unstable Bluffs in Puget Sound, Washington. Coastal Erosion Management Studies. Volume 8. Shorelands and Water Resources Program. Washington Department of Ecology, Olympia, Washington.
- Marchent, C., and J. Sherlock. 1984. A Guide to Selection and Propagation of Some Native Woody Species for Land Rehabilitation in British Columbia. Research Report RR84007-HW. British Columbia Ministry of Forests, Victoria, B.C.
- McCullah, J. and A. L. Kendrick. 1996. Biotechnical Erosion Control for Slopes and Streambanks. Manual prepared for International Erosion Control Association, Professional Dev. Short Course.
- Menashe, E. 1998. Vegetation and Erosion: A literature Survey. In: Proceedings of the Native Plants Symposium, Oregon State University, Forestry Sciences Lab., Corvallis, OR. 1998 Dec. 9-10: 130-135.
- Menashe, E. 1993. Vegetation Management: A Guide for Puget Sound Bluff Property Owners. Shorelands and Coastal Zone Management Program, Washington Department of Ecology, Olympia, Washington.
- Rahn, P. H. 1969. The Relationship Between Natural Forested Slopes and Angles of Repose for Sand and Gravel. Geol. Soc. Am. Bull. 80(10):2123-2128.
- Sangrey, D. A., et al. 1985. Reducing Losses from Landsliding in the United States. Committee on Ground Failure Hazards. Commission on Engineering and Technical Systems. National Research Council. National Academy Press, Washington, D. C.
- Schiectl, H. 1980. Bioengineering for Land Reclamation and Conservation. University of Alberta Press, Edmonton, Alberta, Canada.
- Schueler, T., 1987. Controlling Urban Runoff: A Practical Manual for Planning and Designing Urban BMP’s. Metropolitan Washington Council of Governments, Washington, DC.
- Sidle, R. C. 1985. Factors Affecting the Stability of Slopes. In: Proceedings of a Workshop on Slope Stability: Problems and Solutions in Forest Management, U.S.D.A. Forest Service, PNW Research Station, Portland, Oregon.
- Sidle, R. C., et. al. 1985. Hillslope Stability and Land Use. Water Resources Monograph Series, II. American Geophysical Union, Washington, D. C.
- Sidle, R. C. 1980. Slope Stability on Forest Land. PNW 209. Pacific Northwest Extension. United States Department of Agriculture, Forest Service.
- Stembridge, J. E., Jr. 1979. Beach Protection Properties of Accumulated Driftwood. In: Proceedings of the Specialty Conference on Coastal Structures 79. ASCE/Alexandria, Virginia. 1979 March 14-16:1052-1068.
- Terich, T. A. and S. Milne, 1977. The Effects of Wood Debris and Drift Logs on Estuarine Beaches of North Puget Sound. Dept. of Geography and Regional Planning, Western Washington University.
- Van Dersal, W. 1938. Native Woody Plants of the United Sates, Their Erosion Control and Wildlife Value. USDA, Misc. Pub. No. 303. Washington D.C.
- Wilford, D.J. 1982. The Sediment Storage Function of Large Organic Debris at the Base of Unstable Slopes. In: Proceedings of Old-Growth Symposium, Juneau, Alaska.
- Wischmeier, W. H. 1975. Estimating the Soil Loss Equation’s Cover and Management Factor for Undisturbed Areas. In: Proceedings, Sediment Yield Workshop, Oxford, Miss-ARS-40. New Orleans, LA: U.S.D.A., Agricultural Research Service, Southern Region. Pg. 118-124.
- Wischmeier, W. H., and D. D. Smith. 1978. Predicting Rainfall Erosion Losses – A Guide to Conservation Planning. Agriculture Handbook. No. 537. U.S. Department of Agriculture, Washington, D. C.
- Woods, J. B. 1938. Ligneous Plants for Erosion Control. M. F. Thesis, College of Forestry, University of Washington, Seattle, Washington.
- Zeimer, R. R. 1981. Roots and The Stability of Forested Slopes. International Association of Hydrogeological Sciences. Publication No. 132. pp. 343-361.
- Zelo, I. and H. Shipman. 2000. Alternative Bank Protection Methods for Puget Sound Shorelines. Pub. 00-06-012. Prepared for: Shorelands and Environmental Assistance Program, Washington State Department of Ecology, Olympia, WA.